December 16, 2020
How to transport microwave quantum information via optical fiber
William Hease and Georg Arnold from the Fink group present two transducers that could enable quantum communication between superconducting processors
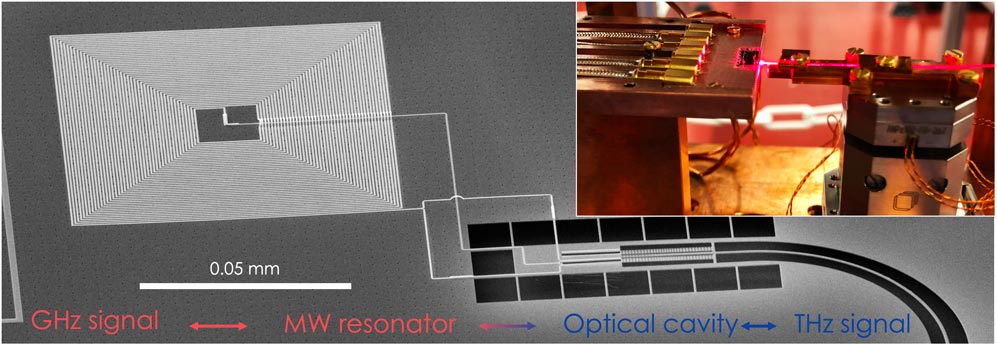
© Georg Arnold / IST Austria
Quantum computing could revolutionize the world with absolutely secure communication and more powerful calculations. Currently, exclusively scientists and tech-giants use quantum computers due to their demanding operating conditions. However, if one could connect multiple quantum machines to a network, a larger audience could start working in the quantum world. Networks would enable distributed & blind quantum computing via secure connections, making quantum computers accessible to the outside. Additionally, basic research would leap forward with an interconnected world of quantum physical systems. However, that connectivity is one obstacle currently blocking the quantum revolution.
Quantum systems are extremely susceptible to noise, particularly if they are based on superconducting electrical circuits. Therefore, most quantum processors need to run at temperatures close to absolute zero in a vacuum environment. Therefore, such a quantum computer looks more like a refrigerator rather than a personal computer from the outside. Due to these technical challenges and their costs, long-distance networks seem to remain a fiction – at first.
Currently, scientists work hard to transport quantum information via standard communication tools like optical fiber. These can easily carry quantum signals over more than hundred kilometers. Here, the transport is not the actual problem, but the signal’s transformation. Superconducting processors usually operate at microwave frequencies where quantum signals are extremely weak compared to ambient noise. Therefore, a perfect transducer would convert signals between the electrical and optical domain without noise or loss.
“Such transducers are ubiquitous in classical communication networks, and they operate with incredible bandwidth and reliability. However, in quantum-level signals, their efficiency needs to be orders of magnitude higher. At the same time, we need to suppress their electronic noise by orders of magnitude,” explains Professor Johannes Fink.
At IST Austria, Professor Johannes Fink and his team investigate quantum networks. Two scientists from his group now approached the transformation of microwave quantum information from different angles. One offering remarkably noise-free signals. The other showing some of the highest conversion efficiencies of any microwave-optics transducer to date.
A micromechanical transducer
Georg Arnold (Fink group) shows the first on-chip microwave-optics transducer based on a mechanical intermediary, which achieves high efficiency at unprecedently low input powers. The scientists designed, fabricated, and tested a new type of hybrid device that works at ultracold temperatures and combines the best aspects of various new technologies.
Georg Arnold summarizes his finding: “We created an integrated link between light and microwave signals with a core size of 0.1×0.2 mm² by making use of the compact size and versatile coupling of mechanical elements to both domains. We use industry-standard fabrication for our prototypes, which gives us high hopes that an optimized version will find a future application. A remaining challenge is local heating due to its minimal dimensions when operating the transducer at its maximum efficiency. While this is typically not an issue for classical signals, it is currently an obstacle for the noise-free transduction required for pristine quantum signals.”
Motion, or more precise vibrations, can connect light, electronic signals, and even more complex physical systems with one another. For the transducer, the scientists use a phenomenon called radiation pressure to transform microwaves to optical light. Radiation pressure means that under certain conditions, light can actually push and pull physical objects.
Within the device’s heart sits a photonic crystal cavity connected to two ultra-thin silicon strings. The end of each line is either a microwave or a fiber input. Like playing a string instrument, the push of incoming light shakes the silicon wires. The whole cavity resonates, and the vibration travels from one end to the other, transforming into the microwave signal. An optical input converts into a microwave signal and vice versa.
“The device essentially acts as a very sensitive mirror that is displaced by the impinging light. Thereby it couples mechanical motion and optical radiation. We optimized the system so that the smallest unit of light, a single photon, can already excite a mechanical oscillation. The microwave output conceptually works in a similar fashion so that we can transduce optical photons to microwave frequencies by mapping them on an intermediate mechanical oscillator,” says Georg Arnold.
This approach currently also amplifies the signal, an effect unwanted for the faithful conversion of quantum information but potentially useful for more power-efficient optical modulation techniques.
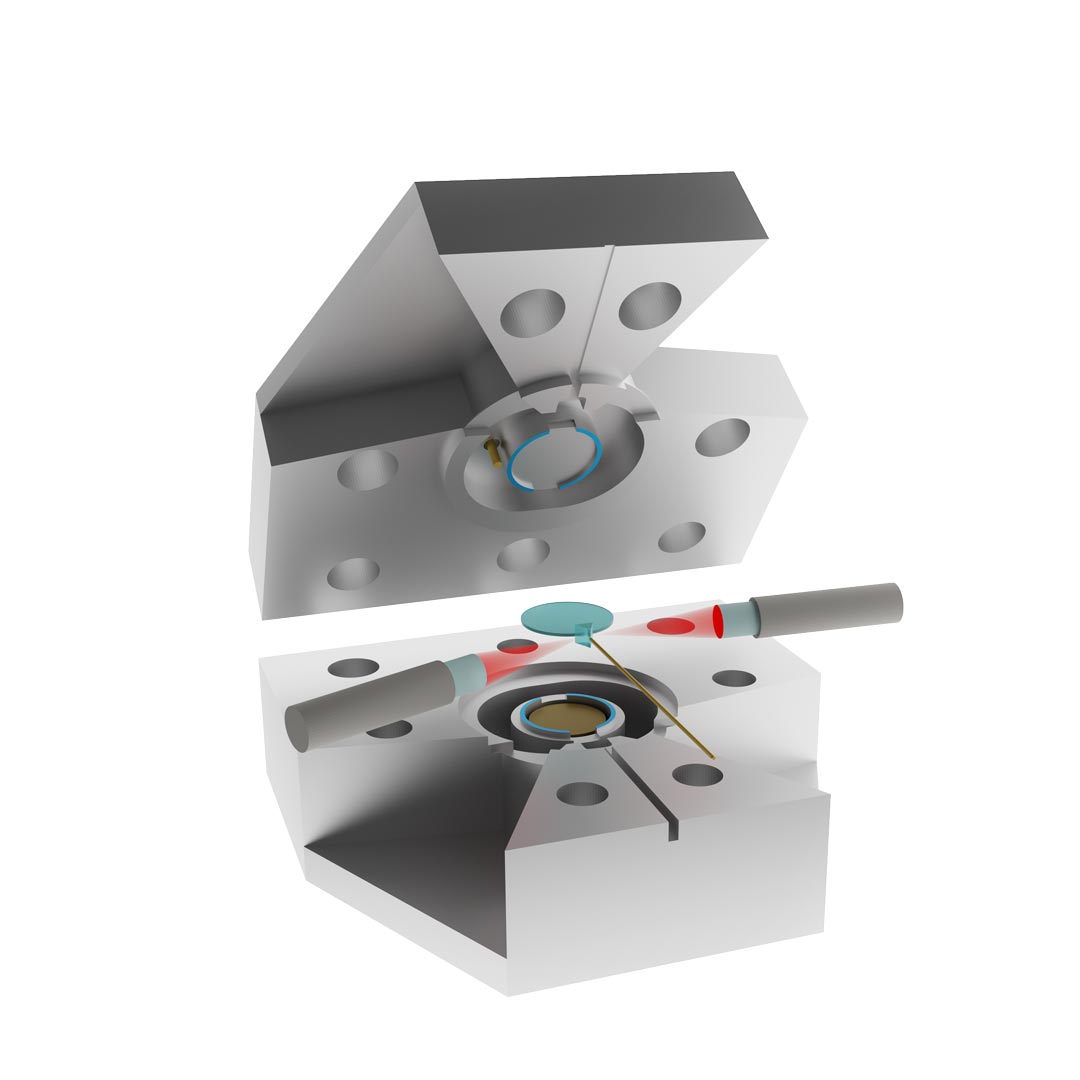
Conversion via optical nonlinearities
William Hease (Fink group) approached the transducer from a different angle. His system transforms the signals as clean as possible and without adding any noise. He took an approach that works strikingly similar to a laser.
“Our approach harnesses the potential of single crystal resonators, in which the light can resonate for thousands of turns before undergoing absorption. This enhances the interaction of light with microwave quantum signals. Additionally, our system’s macroscopic size (~ 1 cm3) makes it quite insensitive to heating effects, which allows keeping the noise associated with the conversion process to a minimum,” explains William Hease.
The light of the optical fiber passes through a nonlinear crystal. Such a crystal strongly changes its properties depending on an applied voltage. Here, the voltage is provided by the microwave signal containing the quantum information to be converted. The controlled interaction with the crystal then also influences the passing of optical light. Therefore, finally, the optical light’s frequency and mode shape are controlled by the quantum signal. At the other end, a receiver could translate these changes back into the microwave signal based on the same principle. This is called a transceiver.
William Hease summarizes his findings: “Our system makes use of nonlinear effects in Lithium Niobate to convert microwave signals to optical ones and vice versa. Contrary to amplifiers based on transistor technologies (CMOS, etc.), Lithium Niobate nonlinear effects would lead to an absolutely noiseless conversion if the system could be kept at zero temperature. In real experiments, this is not the case because of optical heating, but we still manage to keep the amount of noise to an extremely low level.”
This approach offers nearly noise-free quantum transduction – a vital requirement for future quantum communication applications.
The future of quantum converter
An ideal quantum signal converter transforms signals in both directions, from quantum to optical and vice versa, without signal loss or noise addition. Both results from the Fink group try to tackle this challenge in their way. Georg’s micro-mechanical solution offers unprecedented efficiency in an extremely small package and is, therefore, scalable. On the other hand, William’s nonlinear optics approach allows for immaculate conversion with comparable high bandwidth.
Professor Johannes Fink on these two findings “It is exciting to have two strong contenders in the race for a future quantum interface for superconducting processors. While there is still a lot of work ahead, it also starts to become clear that we will be able to perform first basic quantum communication protocols in the next few years.”
Publications
G. Arnold, M. Wulf, S. Barzanjeh, E. S. Redchenko, A. Rueda, W. J. Hease, F. Hassani, and J. M. Fink. 2020. Converting microwave and telecom photons with a silicon photonic nanomechanical interface. Nature Communications. DOI: 10.1038/s41467-020-18269-z
William Hease, Alfredo Rueda, Rishabh Sahu, Matthias Wulf, Georg Arnold, Harald G. L. Schwefel, and Johannes M. Fink. 2020. Bidirectional Electro-Optic Wavelength Conversion in the Quantum Ground State. PRX Quantum. DOI: 10.1103/PRXQuantum.1.020315