May 19, 2023
Wiring up Quantum Circuits with Light
ISTA researchers entangled microwave and optical photons for the first time
The number of qubits in superconducting quantum computers has risen rapidly during the last years, but further growth is limited by the need for ultra-cold operating temperatures. Connecting several smaller processors could create larger, more computationally powerful quantum computers, however doing so poses new challenges. A team of researchers led by Rishabh Sahu, Liu Qiu, and Johannes Fink from the Institute of Science and Technology Austria (ISTA) have now, for the first time, demonstrated quantum entanglement between optical and microwave photons that could lay the foundation for such a future quantum network.
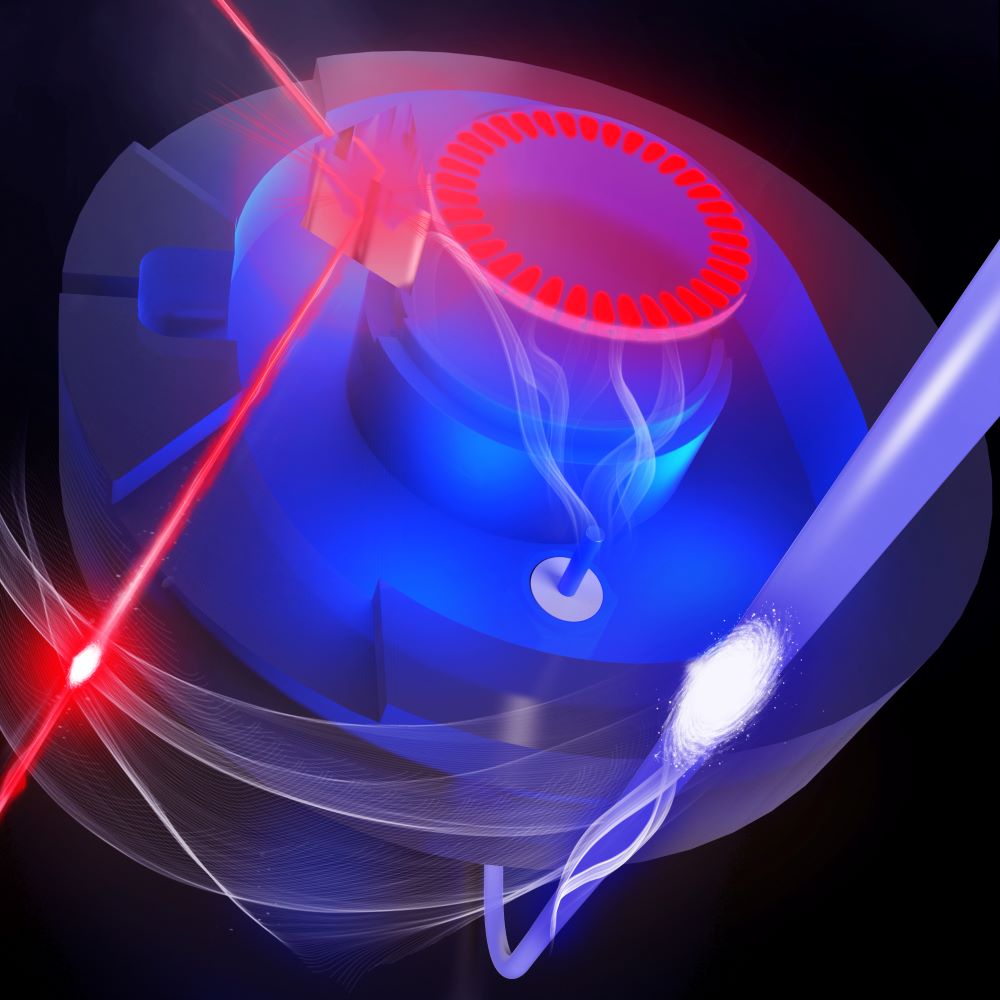
Quantum computers promise to solve challenging tasks in material science and cryptography that will remain out of reach even for the most powerful conventional supercomputers in the future. Yet, this will likely require millions of high-quality qubits due to the required error correction.
Progress in superconducting processors advances quickly with a current qubit count in the few hundreds. The advantages of this technology are the fast computing speed and its compatibility with microchip fabrication, but the need for ultra-cold temperatures ultimately confines the processor in size and prevents any physical access once it is cooled down.
A modular quantum computer with multiple separately cooled processor nodes could solve this. However, single microwave photons—the particles of light that are the native information carriers between superconducting qubits within the processors—are not suitable to be sent through a room temperature environment between the processors. The world at room temperature is bustling with heat, which easily disturbs the microwave photons and their fragile quantum properties like entanglement.
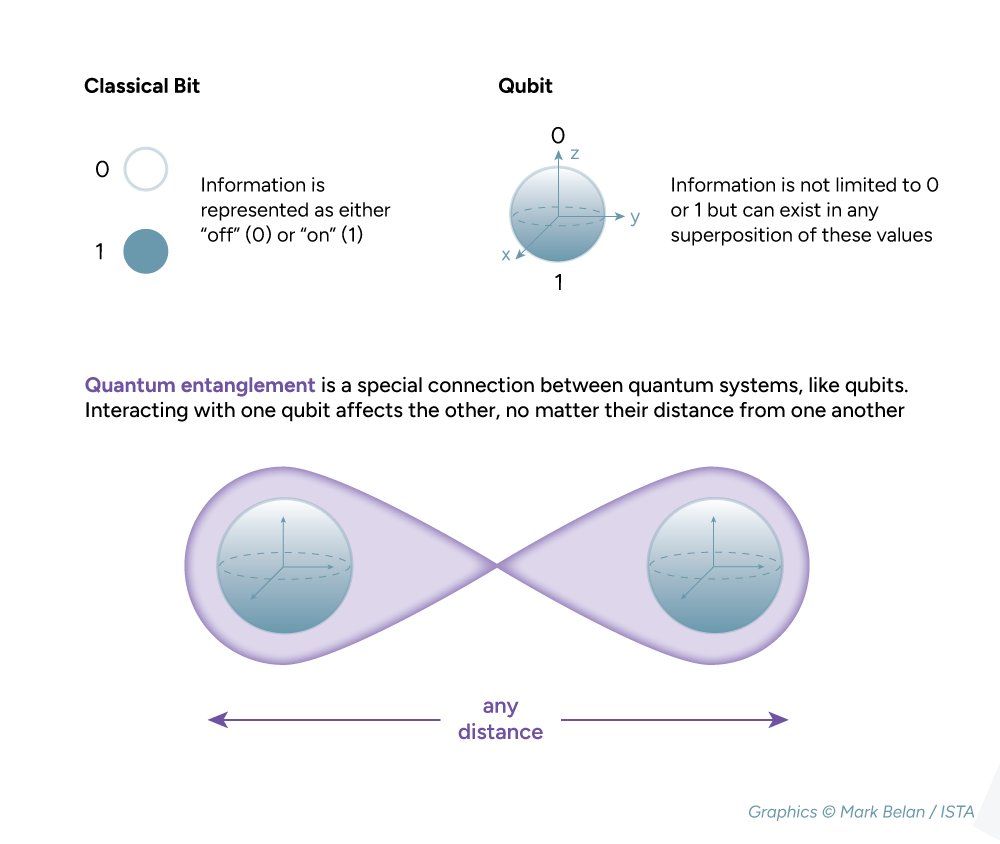
Researchers from the Fink group at the Institute of Science and Technology Austria (ISTA), together with collaborators from TU Wien and the Technical University of Munich, demonstrated an important technological step to overcome these challenges. They entangled low-energy microwave with high-energy optical photons for the very first time. Such an entangled quantum state of two photons is the foundation to wire up superconducting quantum computers via room temperature links. This has implications not only for scaling up existing quantum hardware but it is also needed to realize interconnects to other quantum computing platforms as well as for novel quantum-enhanced remote sensing applications. Their results have been published in the journal Science.
Cooling Away the Noise
Rishabh Sahu, a postdoc in the Fink group and one of the first authors of the new study, explains, “One major problem for any qubit is noise. Noise can be thought of as any disturbance to the qubit. One major source of noise is the heat of the material the qubit is based on.”
Heat causes atoms in a material to jostle around rapidly. This is disruptive to quantum properties like entanglement, and as a result, it would make qubits unsuitable for computation. Therefore, to remain functional, a quantum computer must have its qubits isolated from the environment, cooled to extremely low temperatures, and kept within a vacuum to preserve their quantum properties.
For superconducting qubits, this happens in a special cylindrical device that hangs from the ceiling, called a “dilution refrigerator” in which the “quantum” part of the computation takes place. The qubits at its very bottom are cooled down to only a few thousandths of a degree above absolute zero temperature—at about -273 degrees Celsius. Sahu excitedly adds, “This makes these fridges in our labs the coldest locations in the whole universe, even colder than space itself.”
The refrigerator has to continuously cool the qubits but the more qubits and associated control wiring are added, the more heat is generated and the harder it is to keep the quantum computer cool. “The scientific community predicts that at around 1,000 superconducting qubits in a single quantum computer, we reach the limits of cooling,” Sahu cautions. “Just scaling up is not a sustainable solution to construct more powerful quantum computers.”
Fink adds, “Larger machines are in development but each assembly and cooldown then becomes comparable to a rocket launch, where you only find out about problems once the processor is cold and without the ability to intervene and correct such problems.”
Quantum Waves
“If a dilution fridge cannot sufficiently cool more than a thousand superconducting qubits at once, we need to link several smaller quantum computers to work together,” Liu Qiu, postdoc in the Fink group and another first author of the new study, explains. “We would need a quantum network.”
Linking together two superconducting quantum computers, each with its own dilution refrigerator, is not as straightforward as connecting them with an electrical cable. The connection needs special consideration to preserve the quantum nature of the qubits.
Superconducting qubits work with tiny electrical currents that move back and forth in a circuit at frequencies about ten billion times per second. They interact using microwave photons—particles of light. Their frequencies are similar to the ones used by cellphones.
The problem is that even a small amount of heat would easily disturb single microwave photons and their quantum properties needed to connect the qubits in two separate quantum computers. When passing through a cable outside the refrigerator, the heat of the environment would render them useless.
“Instead of the noise-prone microwave photons that we need to do the computations within the quantum computer, we want to use optical photons with much higher frequencies similar to visible light to network quantum computers together,” Qiu explains. These optical photons are the same kind sent through optical fibers that deliver high-speed internet to our homes. This technology is well understood and much less susceptible to noise from heat. Qiu adds, “The challenge was how to have the microwave photons interact with the optical photons and how to entangle them.”
Splitting Light
In their new study, the researchers used a special electro-optic device: an optical resonator made from a nonlinear crystal, which changes its optical properties in the presence of an electric field. A superconducting cavity houses this crystal and enhances this interaction.
Sahu and Qiu used a laser to send billions of optical photons into the electro-optic crystal for a fraction of a microsecond. In this way, one optical photon splits into a pair of new entangled photons: an optical one with only slightly less energy than the original one and a microwave photon with much lower energy.
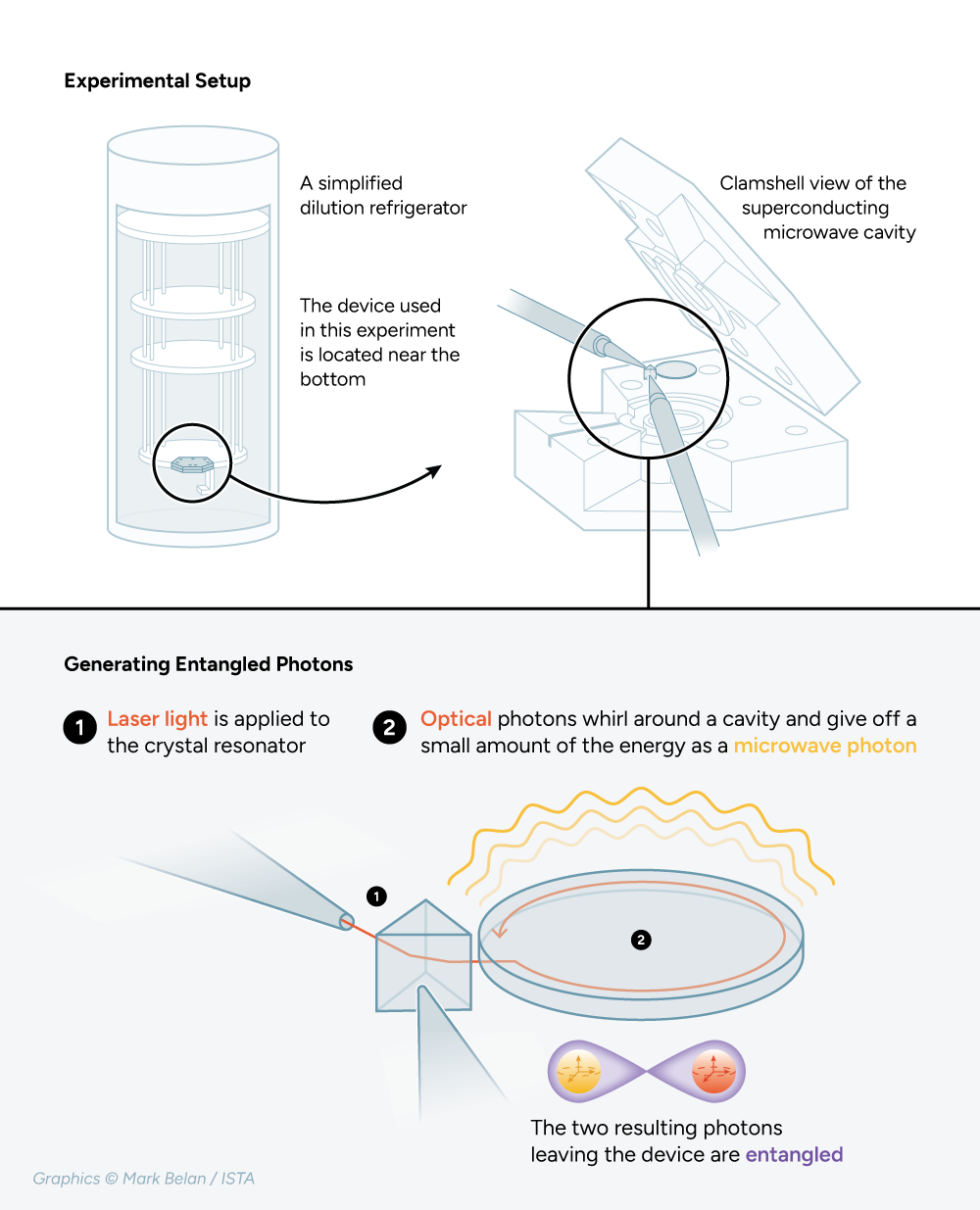
“The challenge of this experiment was that the optical photons have about 20,000 times more energy than the microwave photons,” Sahu explains, “and they bring a lot of energy and therefore heat into the device that can then destroy the quantum properties of the microwave photons. We have worked for months tweaking the experiment and getting the right measurements.” To solve this problem, the researchers built a bulkier superconducting device compared to previous attempts. This not only avoids a breakdown of superconductivity, but it also helps to cool the device more effectively and to keep it cold during the short timescales of the optical laser pulses.
“The breakthrough is that the two photons leaving the device—the optical and the microwave photon—are entangled,” Qiu explains. “This has been verified by measuring correlations between the quantum fluctuations of the electromagnetic fields of the two photons that are stronger than can be explained by classical physics.”
“We are now the first to entangle photons of such vastly different energy scales.” Fink says, “This is a key step to creating a quantum network and also useful for other quantum technologies, such as quantum-enhanced sensing.”
Publication:
R. Sahu, L. Qiu, W. Hease, G. Arnold, Y. Minoguchi, P. Rabl, J. M. Fink. 2023. Entangling microwaves with light. Science. DOI: 10.1126/science.adg3812
Funding information:
The project was supported by the European Research Council (grant no. 758053, ERC StG QUNNECT), the European Union’s Horizon 2020 Research and Innovation Program (grant no. 899354, FETopen SuperQuLAN; grant no. 862644, FETopen QUARTET; Marie Sklodowska-Curie grant no. 754411), the ISTFELLOW program, the Austrian Academy of Sciences, and the Austrian Science Fund (FWF) through BeyondC (grant no. F7105).